
Introduction
The Internet of Medical Things (IoMT) encompasses a diverse range of wireless technologies serving various health care applications. These include enterprise Wi-Fi for hospital environments with challenges with radio frequency connectivity, zero provisioning support for home health care applications, Bluetooth options for seamless interfacing with numerous smartphones and tablets, and connectivity solutions for cost-effective sensor deployment and wire replacement.
It’s possible to connect almost anything to the internet: devices, machines, buildings, and a host of other products. Such connectivity creates numerous benefits and opportunities. With a wide range of advanced wireless technologies and protocols like Bluetooth, Wi-Fi, LoRa, and cellular at your disposal, how do you decide where to begin? How do you select the right connectivity option for your medical devices and IoMT applications?
As a hospital or medical device company decision-maker, it’s important that you understand not only the concept of IoMT, but also market dynamics in the current health care environment and the best type of connectivity for your particular situation.
As a hospital or medical device company decision-maker, it’s important that you understand not only the concept of IoMT, but also market dynamics in the current health care environment and the best type of connectivity for your particular situation.
Market dynamics in health care
In the health care industry, it’s crucial that patient health data is precise, available, securely stored, securely transferred, and captured almost instantaneously. The market demands intelligent devices that can seamlessly integrate with existing health care systems, including electronic medical records (EMR), billing, and asset management, to enhance data accuracy and workflow efficiencies. As a result, wireless connectivity is being integrated into a growing number of devices, and patients are wearing wireless sensors to monitor their health and environmental conditions. Health care providers in acute, clinical, and home health care settings are increasingly relying on IoMT technology to improve workflow efficiencies, enhance patient treatment, and reduce costs.
Within the IoMT ecosystem, intelligent devices gather and transmit crucial data in real time. The potential uses of this technology for advancing health care on a global scale are innumerable.
For example, let’s say a patient is a teenager with diabetes who is struggling to manage their blood glucose levels and experiencing frequent and extremely high or low readings. Rather than scheduling an appointment, the doctor can download the patient’s glucose readings remotely from their connected continuous glucose monitor system. This allows real-time analysis and diagnostics for the patient instead of having to rely on an in-patient visit to react to changing conditions.
It’s important to understand the market conditions of the health care environment before you make crucial decisions about connecting your medical devices to IoMT applications. There are a few market conditions that continue to drive the development and deployment of the IoMT.
There are numerous ways the IoMT can aid in health care:
|
|
Connectivity is what brings these four market dynamics — data, cloud analytics, innovation, and mobility — together, successfully connecting people and devices to the health care network no matter the medical purpose or location of the connected device.
Data
The volume of medical knowledge and data is rapidly increasing. It took 50 years for medical data to double in 1950, but as of 2023, it only takes around 70 days to double. Health care providers are now required to use electronic medical records to monitor and maintain patients’ medical data. The use of wireless technologies is automating data collection from patients throughout the care continuum. Additionally, connected devices are increasingly being utilized for asset tracking, predictive maintenance, and environmental monitoring. Asset-tracking and inventory management solutions are projected to double in the next few years. These efforts ensure that medical equipment is being used effectively and that temperature-sensitive devices and materials are being properly maintained.
Cloud analytics
As innovation continues to grow rapidly and connected technologies produce increasingly large amounts of data, more complex systems are necessary to collect, store, and process this information. Without efficient means of gathering, maintaining, and analyzing data, its abundance becomes useless in health care settings, where it can be used for diagnosis, treatment, and improved efficiency. The growth of analytics and Blockchain technologies will play a vital role in achieving rapid and accurate diagnostics. These technologies will also facilitate data exchange and improved care by connecting electronic medical records, enabling seamless sharing of records.
Innovation
At present, medical device innovation is outpacing pharmaceutical advancements, with personal monitoring devices like fitness trackers and continuous glucose monitoring systems experiencing a surge in popularity. This trend is expected to continue in both acute care and home health care settings. Patient monitoring devices, such as pumps, are becoming smaller and wireless, freeing patients from being tethered to fixed locations. The use of wireless technologies and robotic surgeries in the operating room is also on the rise. The accelerated pace of innovation, coupled with the growth of IoMT technologies and applications, is placing greater pressure on health care providers to adopt these advancements in order to improve patient care and satisfaction while also reducing costs.
Mobility
Health care providers are in constant motion, and mobile connectivity is critical to their daily operations. Health care devices (and therefore health care services) are expanding beyond the secure environment of hospitals and doctor’s offices, increasing mobility to support improved patient quality of life. This expansion increases the need for strong, reliable wireless connectivity.
Understanding connectivity technologies
When it comes to the IoMT, there are three primary connectivity options that merit careful consideration: Wi-Fi, Bluetooth, and low-power wide-area network (LPWAN).
There are two ways to incorporate connectivity into devices for the IoMT. It can either be embedded in the device during manufacturing or added externally after the device has been deployed. Current market expectations are that new smart devices should already have connectivity integrated into them.
To collect data on a local level, gateways can be utilized. These gateways can then manage the connection to a cloud server for the transmission of the data. This approach eliminates the need for every device or sensor to be connected to a cloud or network server. The following sections provide an overview of the wireless technologies used for connectivity in IoMT devices, along with important aspects and considerations for each.
Enterprise Wi-Fi
Wi-Fi uses radio waves to transmit information at specific frequencies. It enables high-speed and secure communication over both short and long distances, making it suitable for deployment within enterprises. However, it’s important to note that we are discussing enterprise-grade Wi-Fi, which offers superior performance, security, compliance with standards, and life-cycle management as compared to consumer-grade Wi-Fi.
In acute care settings such as hospitals and ambulatory care settings like clinical facilities, enterprise Wi-Fi is the preferred choice for local area networks. Fast and secure roaming is a critical performance criterion in these environments, as network connectivity interruptions during device movement can result in data loss and delays in administering health care to patients. As such, it is imperative for Wi-Fi modules to support optimized scanning algorithms that ensure network persistence for mobile devices even in noisy radio frequency environments.
Enterprise Wi-Fi technology operates on two communication bands: 2.4 GHz and 5 GHz. When deploying devices, it is important to consider which band they will be transmitting on. Due to the potential congestion caused by commodity devices and guest access, many hospitals designate their 5 GHz band specifically for critical devices and applications. This is because the 5 GHz band offers increased bandwidth and performance compared to the 2.4 GHz band. To gain a better understanding of this, let’s delve into the latest Wi-Fi standards in more detail.
802.11ac: The standard of choice for enterprise Wi-Fi
If you’re considering embedded Wi-Fi for your device, the recommended standard to pursue is 802.11ac Wave 2, also known as Wi-Fi 5. Although not an official IEEE standard, Wave 2 offers improved performance and additional features compared to 802.11ac Wave 1, such as multiple user-multiple input, multiple output (MU-MIMO) capabilities. Notably, 802.11ac introduces significant enhancements to the Wi-Fi standard, including 80 MHz/160 MHz channels and MU-MIMO capabilities.
One of the advantages of 802.11ac is that it operates in the less-congested 5 GHz signal range, reducing interference commonly found in the 2.4 GHz range and allowing for wider channel implementation. Moreover, 802.11ac devices are backward compatible with previous 802.11a and 802.11n 5 GHz devices. Additionally, most 802.11ac dual-band access points also support 802.11b/g/n in the 2.4 GHz band.
80 MHz and 160 MHz channels
The enhanced performance and bandwidth of 802.11ac are primarily driven by its support for larger channel widths, including 80 MHz and 160 MHz. In contrast to the previous method of denoting 5 GHz channels by using the 20 MHz center channel frequencies for both 20- and 40-MHz-wide channels, 802.11ac now references the center frequency for 20-, 40-, 80-, or 160-MHz-wide channels. This results in specific channel numbers for each channel width, as shown in figure A.
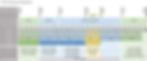
Source: Wireless LAN Professionals
With wider channels come higher data rates and bandwidth. But careful channel planning must take place because these wider channels come at a cost. It’s possible that these wider channel widths may not be realistic in an enterprise Wi-Fi environment where multiple access points are deployed and co-channel interference must be avoided. As you can see in figure A, there is only one 160 MHz channel available in the United States and two in the European Union (if dynamic frequency selection is used). With the 80-MHz-wide channels, four or five are available, depending on where you are located. To reap the benefits of improved performance from these wider channels, thorough channel planning must take place to avoid co-channel interference.
MU–MIMO
MU-MIMO is a technology that enhances the support for wireless networks with multiple users. With the earlier single-user MIMO technology, access points could only send data to one device at a time which caused congestion. However, with MU-MIMO, access points can send downstream traffic to up to four clients simultaneously (see figure B).

The benefits of MU-MIMO technology include reduced on-air time for multiple clients, significantly improved overall network efficiency, and enhanced transfer of large files and video streaming. For devices to benefit from MU-MIMO technology, both the access point and the clients must support it. That being said, even non-MIMO devices could experience some improved performance; if MU-MIMO devices on the same network are served more quickly, there is likely more time for single user-MIMO and other devices to communicate.
802.11ax
The next-generation 802.11ax Wi-Fi standard, also known as Wi-Fi 6, is the latest step in a journey of nonstop innovation. The standard builds on the strengths of 802.11ac, adding efficiency, flexibility, and scalability that allows new and existing networks increased speed and capacity with next-generation applications. The latest specification provides higher capacity and better coverage, reducing congestion for a better user experience.
Some features and benefits of 802.11ax include the following:
802.11ax builds on the advantages of 802.11ac by allowing even more simultaneous transmissions. While 802.11ac supports up to four downlink transmissions at a time, 802.11ax supports up to eight transmissions both for downlink and uplink.
OFDMA is a new feature that allows access points to send multiple packets to multiple users at the same time. In contrast, previous specifications only allowed one packet to be sent per client using OFDM. By using OFDMA, a single packet can now serve multiple clients, as the channel is divided into numerous smaller sub-channels. With up to 30 clients sharing each channel, there is no need for them to take turns broadcasting and listening, thus improving network efficiency.
The 802.11ax standard utilizes 1024-QAM modulation, enabling devices to send denser packets and thus facilitating the transmission of more data per packet.
Resource scheduling: This new feature is a more efficient way to handle packets. It significantly increases a device’s sleep time, improving battery life. Think of it like a traffic light at an intersection, allowing cars to flow without congestion and in an orderly manner.
Backward compatibility: 802.11ax is backward compatible with 802.11a/b/g/n/ac.
Increased spectrum use: 802.11ax provides better coverage, operating in both the 2.4 GHz and 5 GHz frequency bands, while 802.11ac only works in 5 GHz.
Enterprise Wi-Fi security
Obviously, when dealing with health care and the IoMT, secure communication is vital. Wi-Fi data security involves two key elements: encryption and authentication. Encryption scrambles data so it cannot be intercepted, and authentication verifies that the client receiving the data is the client who should be receiving the data.
The three primary security algorithms for wireless networks are Wired Equivalent Privacy (WEP), Wi-Fi Protected Access (WPA), and Wi-Fi Protected Access II (WPA2). WEP is the oldest and has proven to be vulnerable to security breaches as more flaws have been uncovered. While WPA improved security, it is also now considered to be susceptible to intrusion. WPA2 is currently the most secure choice available, although it is not without flaws. When securing networks with WPA2, you will see two different types of encryption: Temporal Key Integrity Protocol and Advanced Encryption Standard (AES). AES, which is a more secure encryption protocol, was introduced with WPA2. Beyond just a Wi-Fi standard, it is a globally recognized encryption standard that has been adopted by the US Government.
Extensible Authentication Protocol (EAP) is a framework used for authentication that provides a set of common functions and the ability to negotiate authentication methods (called EAP methods). There are approximately 40 different EAP methods currently defined, and some of the most commonly used modern methods that can operate in wireless networks include EAP-TLS, EAP-SIM, EAP-AKA, LEAP, and EAP-TTLS.
WPA2-Pre-Shared Key (PSK) versus WPA2-Enterprise
Below is a brief comparison of the less secure WPA2-PSK and WPA2-Enterprise.
WPA2-PSK | WPA2-Enterprise |
---|---|
|
|
The security protocol used in a Wi-Fi network is determined by the IT organization of the end user. In the medical market, most enterprise networks require support for WPA2-Enterprise for clients joining the network. The choice of EAP supplicant is also driven by the end user and the selected RADIUS authentication method. For medical device manufacturers, the challenge lies in implementing a Wi-Fi communications module that supports WPA2-AES encryption and provides updated EAP supplicant drivers.
WPA3
In January 2018, the Wi-Fi Alliance announced Wi-Fi-certified WPA3, the next generation of Wi-Fi security for both personal and enterprise networks. This newest version adds four features that were not present in WPA2. To market devices as Wi-Fi Certified™ WPA3™, manufacturers must fully implement these four new features:
Individualized data encryption: When you connect to an open Wi-Fi network (such as in a coffee shop or airport), the traffic between your device and the access points is encrypted even though no password was entered during the connection process.
New handshake: When a device connects to an access point, it performs a handshake to ensure you’ve used the correct password to connect and negotiates the applicable encryption that will secure the connection. This new handshake delivers stronger protections even if the user assigns a password that doesn’t meet typical strength recommendations.
Simpler connection process: Because many devices today do not have displays, WPA3 includes a feature that simplifies the security configuration process.
192-bit security suite: Intended for government, defense, and industrial applications, this suite aligns with the Commercial National Security Algorithm Suite from the Committee on National Security Systems.
A number of Wi-Fi solutions available today can support a software migration to WPA3. The exception is support for 192-bit encryption. This may require a hardware upgrade for some Wi-Fi modules and chipsets.
Not sure about what technology to use in your particular case? See if we can help you. Contact Us.
Bluetooth for health care
At first, health care organizations were hesitant to embrace Bluetooth because of concerns about adding another radio frequency technology in an already crowded wireless environment. However, as Bluetooth security has improved and signal range has increased, more and more medical applications are using Bluetooth. The integration of Bluetooth in smartphones and tablets has significantly increased its adoption. Since Bluetooth uses frequency-hopping technology, it can avoid channels in the spectrum that are congested with other wireless technologies like Wi-Fi access points.
Bluetooth is increasingly utilized in the medical industry for a variety of purposes. Personal monitoring devices such as fitness trackers, glucose monitors, and neuromodulation tools often rely on Bluetooth for connectivity and provisioning. In critical care environments, there is an increase in the use of these types of monitoring devices. Additionally, Bluetooth is being used as a wire replacement in operating rooms, intensive care units, and other areas with a high number of devices. Finally, Bluetooth can be used to aid patients and visitors with wayfinding, providing a customer service benefit.
Understanding Bluetooth technology
Bluetooth is a wireless technology that allows mobile Bluetooth devices to exchange data over short distances. The original Classic Bluetooth was designed to continuously stream data over short distances. To put it simply, you can exchange a lot of data with Bluetooth as long as it’s exchanged at close range.
The more recently developed Bluetooth Low Energy, also known as BLE or Bluetooth LE and introduced in Bluetooth 4.0, is a low-power yet robust technology intended for situations where battery life is more important than high data transfer speeds. Although it far exceeds Classic Bluetooth on many fronts, the two are similar in a variety of ways.
They are both WPAN standards that operate in the 2.4 GHz frequency band.
They both operate in a basic master–slave model where both Bluetooth devices must be paired before they can transmit data.
Both use the same pairing, authentication, and encryption technologies.
The key distinction between the two technologies is that BLE consumes significantly less power than Classic Bluetooth. This makes BLE ideal for applications that intermittently send small amounts of data, which is common in the health care field for devices such as blood glucose monitors, pumps, asthma inhalers, and implantables like pacemakers and cardioverter defibrillators. The low-power properties of BLE also make the technology suitable for deploying environmental sensors and room monitoring to meet compliance requirements. BLE is a practical connectivity choice for distributed gateways that collect this data and transmit it to cloud and server applications for analysis.
The following table summarizes the differences between Classic Bluetooth and the much-preferred Bluetooth Low Energy
Technology | Optimized for | Data rate (max.) | Frequency band | Range | Security | Typical use examples |
---|---|---|---|---|---|---|
Classic Bluetooth (BR/EDR) | Continuous data streaming | Up to 3 Mbps | 2.4 GHz | Up to 30 meters | Secure Simple Pairing |
|
Bluetooth (LE) | Short-burst data transmission | 1 Mbps | 2.4 GHz | Up to 150 meters | LE Secure connections |
|
Bluetooth 4.0 entered the technology market in 2011. Since that time, additional versions, including 4.2, 5.0, and 5.1, have been released. The following summarizes some of the enhancements each of these technology versions provide.
Bluetooth 4.2 |
|
---|---|
Bluetooth 5.0 |
|
Bluetooth 5.1 |
|
Bluetooth mesh network
With the advancement of technology and increasing reliance on wireless networks, the demands placed on these networks continue to escalate. Bluetooth mesh, introduced in 2017, is a networking technology that replaces the traditional one-to-one Bluetooth connection with a many-to-many relationship between Bluetooth devices.
In general, mesh networks are capable of efficiently meeting communication requirements over large areas while monitoring and managing multiple devices. Bluetooth mesh networking, in particular, achieves these objectives while also maintaining compatibility with current computers, tablets, and smartphones and leveraging the low-energy efficiency of Bluetooth LE technology.
Bluetooth mesh technology has the potential to improve the deployment of large device networks. For instance, in a hospital setting, a Bluetooth mesh network can aid in monitoring patients, equipment, and staff from any device located on the premises.

Traditional networking methods may not be able to connect the e
ntire hospital effectively or consistently due to numerous obstacles such as walls, electronic equipment, and people. Bluetooth mesh networking enables some devices to act as relays, receiving and retransmitting messages from other devices, allowing communication even with devices that are not within their radio range.
The managed flooding technique employed by mesh network technology is highly effective. A mesh node sends out data in all directions, flooding all nodes within its range. Each of these nodes does the same, flooding all nodes within their respective range. This process repeats until the published data reaches all nodes within the network. Only nodes that are addressed or subscribed to the data act on this transmitted information, while the rest simply relay the messages.
Bluetooth mesh networking is an exceptionally reliable system, as it does not rely on a centralized router and provides multiple available paths for a published message. It also has self-healing capabilities while maintaining its low-power feature. Bluetooth mesh enables low-power devices to operate with other nodes that are not power constrained. These non-power-constrained nodes are responsible for storing messages intended for low-power nodes and only deliver the messages when the low-power nodes request them, thus reducing the energy consumption of low-power nodes.
Low-power wide-area network
With the increasing adoption of the IoMT, we are witnessing a growing number of devices that connect to a central application server, typically hosted in the cloud. Enterprise Wi-Fi and Bluetooth are reliable local area network technologies that can utilize the enterprise network to establish a connection to the cloud. However, there are situations within the enterprise space where direct device connectivity to the enterprise network may not be feasible or may raise security concerns. In such cases, devices and sensors can utilize LPWAN technology to bypass the local network and connect to a carrier network, enabling them to establish a connection back to the cloud.
Using LPWAN protocols, such as LoRa, can enhance the range and propagation of certain applications. Operating in the 900 MHz range, LoRa can provide greater range and better propagation through building materials compared to Wi-Fi or BLE. As a result, LoRa can serve as a cost-effective gateway technology that collects data from distributed sensors and communicates it back to the cloud server from a single fixed point. This allows for low-cost sensor deployment and IoMT across a health care provider’s campus, without the need for sensors to connect to the LAN.
LPWAN technologies, such as LTE CAT-M1 and NB-IoT, are emerging as highly effective solutions for connecting remote sensor devices, including medical devices that are utilized in the home. These protocols differ from standard cellular services in that they are designed to support low data rates at a significantly lower cost compared to standard voice and data cellular services. This enables health care providers to administer patient care within the home while staying seamlessly connected and receiving real-time updates from the point of care. An essential aspect to consider when deploying these technologies in the home is zero provisioning from the patient end. In other words, once the devices are turned on, they automatically establish a connection without requiring any extra steps from the patient to set up the IoMT connection.
LPWAN isn’t just a single technology but a comprehensive term that encompasses a group of protocols that use low-powered devices to transmit small amounts of data over extended distances. Within the LPWAN domain, several technologies are vying for dominance, including LoRa, LoRaWAN, and cellular protocols like LTE CAT-M1 and NB-IoT.
The following table compares basic characteristics and features of LoRaWAN, LTE CAT-M1, and NB-IoT. The following sections provide more in-depth information on each of these technologies.
| LoRaWAN | LTE CAT-M1 | NB-IoT |
---|---|---|---|
Bandwidth (uplink) | 125 kHz | 1,4 MHz | 200 kHz |
Data rate | 290bps - 50Kbps | 1 Mbps | 100 kbps |
Mobility | Yes | Yes | Yes |
LoRaWAN
Long-range wide-area network (LoRaWAN) is a LPWAN media access control layer protocol built on top of LoRa or FSK modulation. LoRaWAN enables low-powered, battery-operated devices to wirelessly communicate over long distances (two to three kilometers in urban settings and six to 10 in rural settings). LoRaWAN can span these extremely long distances by decreasing its data rates to very low levels (0.3–22 kbps).

LoRaWAN is a protocol that operates in the unlicensed ISM band, with the frequency band varying by region. For instance, in the United States, LoRaWAN operates in the 902–928 MHz frequency band, while in Europe, it operates in the 863–870 MHz frequency band.
Of all the LPWAN protocols available, LoRaWAN stands out for its unique ability to be deployed either on a public network or its own private network. Its ability to be deployed on a private LoRaWAN network provides a distinct advantage for several reasons, including the following:
Location: The environment in which you plan to deploy your network may not have a public LoRaWAN network in place.
Remote or inaccessible sensor locations: If you need to deploy sensors in extremely remote or difficult-to-access areas (such as deep basements), a public network may not be accessible.
Large deployment area: If your plan is to deploy a very large number of LoRa sensors, having your own LoRaWAN network can decrease the overall cost.
Security: Health care environments are especially concerned about secure data communications. Avoiding a public LoRaWAN operator and using your own private network heightens the amount of security.
With LoRaWAN, the applications are endless: cold chain monitoring, environmental monitoring, facility security monitoring, monitoring in remote areas, and asset location, just to name a few.
LoRaWAN summary:
Long range: up to 10+ kilometers
Low power: can last years on a battery
Secure: 128-bit end-to-end encryption
Low bandwidth
Inexpensive
For situations where real-time data is not required (since you can only send periodic packets)
Comparing cellular IoMT Technologies: LTE Cat-M1 vs. NB-IoT
Cellular IoMT technologies allow you to connect devices, such as sensors, to the internet via the same mobile networks that smartphones use. Your smartphone uses larger amounts of bandwidth for voice and data. IoT applications usually require a lot less. Cat 1 and Cat M1 modems allow a lot more IoT traffic to fit in the same LTE network bandwidth. LTE Cat 1 uses less network bandwidth than traditional LTE modems, and Cat M1 uses significantly less. Cat 1 supports higher bandwidth (10 Mbps) at a higher power consumption than Cat M1. With a focus on battery-powered devices, the two cellular IoMT technologies we discuss here are LTE Cat-M1 and NB-IoT.
Long-Term Evolution Cat-M1 (LTE Cat-M1) is a low-power, wide-area protocol that allows IoMT devices to connect directly to a 4G network without a gateway. With LTE CAT-M1, you have low bandwidth at a lower cost, long battery life, and lower complexity. LTE Cat-M1 is designed for devices that are moving or require near-real-time speeds.
Narrowband IoT (NB-IoT) is a cellular-grade wireless technology that is intended for extremely low-data-rate devices that must connect to a mobile network. NB-IoT sends and receives small amounts of data. Like LoRaWAN, it is message based, but it has a faster modulation rate, can handle much more data, and has better in-building penetration. NB-IoT provides very low bandwidth with very low power and no roaming capabilities. NB-IoT is perfect for stationary devices and devices that only send updates every couple of minutes.
Based on the comparisons above, the major differences between NB-IoT and LTE-M can be briefed as follows:
| NB-IoT | LTE Cat-M1 |
---|---|---|
Peak data rate | <100 kbps | >384 kbps, up to 1 Mbps |
Latency | 1.5–10 ms | 50–100 ms |
Bandwidth | <200KHz | 1.4 MHz |
Power consumption | Best at very low data rates | Best at medium-to-high data rates |
Mobility | No for Cat-NB1, limited for Cat-NB2 | Yes |
Support for voice (VoLTE) | No | Yes |
Antennas | 1 | 1 |
LTE Cat-M1 and NB-IoT summary comparison
LTE Cat-M1 |
|
---|---|
NB-IoT |
|
Wireless gateways for medical applications
Thus far, we have evaluated several connectivity technologies, with a particular emphasis on connecting individual devices. However, within the realm of IoMT, we are also witnessing a surge in the adoption of gateway technology.
A gateway is a physical device or software program that serves as a connection point between the cloud server/application and devices and/or sensors. All data moving to the cloud, or vice versa, goes through the gateway, which can be either a dedicated hardware appliance or software program.
Smart devices along with environmental, pressure, and positioning sensors can generate thousands of data points per second. A gateway provides a device to collect and preprocess or package the data locally before sending it to the cloud. In this way, the end user can minimize the volume of data needed to be sent and can manage a secure connection through the internet and into the target cloud provider. Because the gateway manages information moving in both directions, it can protect data from leaks and IoT devices from being compromised by malicious outside attacks.
A gateway can support multiple connection technologies, such as Wi-Fi, BLE, LoRa, Ethernet, and serial port connections. These technologies can be used as ingress to the gateway in collecting data from the local devices.
Most deployments use an Ethernet or Wi-Fi connection to the local area network as the doorway to manage the cloud connection. If using the local area network is not feasible, then a cell modem can be integrated into the gateway to leverage cellular connectivity to connect back to the cloud. The LTE CAT-M1 and/or NB-IoT service connections can be a cost-effective egress technology for lower data rate applications. Maintaining a secure connection across all the technologies is critical, specifically for a gateway in use for medical applications or data gathering.
There are several popular cloud service providers available in the market today, including Amazon Web Services, Microsoft Azure, and Google Cloud. These providers offer secure connections to the cloud and enhanced security to protect the data while it is in the cloud. If any patient data is transferred or stored, the cloud service must be HIPAA compliant to meet the privacy security compliance requirements. The previously mentioned providers do offer HIPAA-compliant cloud hosting as an additional feature.
They also offer software connectors, such as Amazon’s Greengrass or Microsoft Azure IoT Edge, that can be run on the gateway to manage secure connections back to the target cloud service. With these connectors, you can use familiar languages and programming models to create and test your device software in the cloud and then deploy it to your devices. The cloud connector can be programmed to filter device data and only transmit necessary information back to the cloud. You can also connect to third-party applications, on-premises software, and other services with cloud connectors. Several gateways can come bundled with the cloud connectors already active. They can also support programming languages, such as Python, to enable fast integration of your software application.
Leveraging cloud connectors and high-level programming languages enables rapid prototyping of applications and collecting and transmitting target data into the cloud. Some applications can be up and running and transferring sensor data into the cloud within minutes.
Gateways accomplish several tasks:
They collect many types of vital information: sensor data, device status and location, serial readouts, and machine status, just to name a few.
They help various sensor protocols and technologies (such as Wi-Fi, Bluetooth, cellular, serial ports, and Ethernet) communicate with one another.
They process the collected data before sending it onto the cloud.
Not only does a gateway provide rugged connectivity with a variety of interfaces, but it also accomplishes this while maintaining high levels of security.
Securing networked medical devices
In addition to network encryption and authentication, there are additional security aspects to consider to ensure the safety of the device from any threats spreading via the network.
Hardware root of trust
Due to the potential security risks associated with IoMT devices, it is crucial to establish a secure and reliable system for their use. Root of trust (RoT) is a term used to describe hardware, firmware, and software components that are highly reliable and perform critical security functions. These components must be secure themselves in order to be trusted. By incorporating RoT within the hardware, only signed and trusted software images are loaded onto the device. This serves as the primary defense against malicious hackers loading suspicious software on the device.
Firewall
Basically, a firewall is a barrier designed to prevent hackers from accessing secured, sensitive, and vital information. By placing a firewall function within your device, it increases the trust level of network connections, and potential attacks can be prevented.
OTA updates
Performing manual security updates to individual IoMT devices can be challenging due to their large scale and distribution. As a solution, over-the-air (OTA) updates can remotely update the software or firmware of connected devices. A reliable OTA update mechanism can help ensure that devices are updated with the latest security fixes, especially for Wi-Fi security, and it’s an efficient way to manage a large number of devices spread out in different locations.
Conclusion
Selecting the optimal connectivity option for your medical device or medical environment, as well as for your IoMT applications, is a complex and critical decision. The deeper your understanding of IoMT concepts, connectivity technologies, market trends, and your own health care environment and requirements, the better equipped you will be to make informed decisions in this regard.